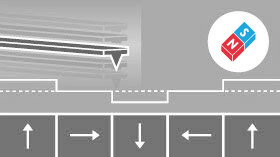
Magnetic Force Microscopy (MFM)
Our MFM mode measures the magnetic variations over a sample surface by detecting the interaction between a magnetized cantilever and sample surface. The cantilever measures surface topography on the first scan, then lifts and follows either the stored surface topography (lift mode, available only in selected countries) or a constant distance (or constant height) at a fixed height above the sample surface.